After recent reports on Red Bull criticising Mercedes car being against the regulations, we look back on last year’s rivalry on their aeroelastic wings. Read our technical spotlight by our writer Ingrid Fazakas.
What were the 2021 regulations?
Last year’s 2021 rules centred on the uniform bending of the rear wing’s structure as a whole, but they are rather focused on the vertical and horizontal movement than the rotational shift of the wing, which was Red Bull’s and many other teams’ case against Mercedes. Vertically, the rear wing is not allowed to deflect more than 3mm under a downward force of 500N. Horizontally, it was illegal for it to move more than 1o under a rearward force of 1000N.
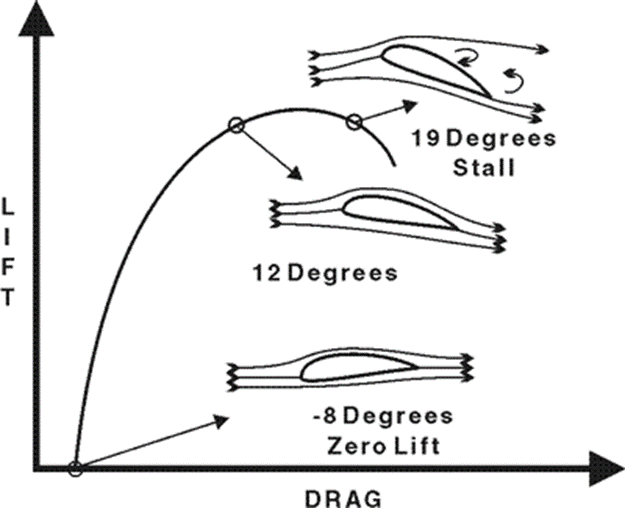
(Source: insideracingtechnology.com)
Mercedes were being accused of using their own flexiwing, however in their case it was the front wing that bent excessively. ‘Any part of the trailing edge of any front wing flap may deflect no more than 5mm, when measured along the loading axis, when a 60N point load is applied normal to the flap’ is what the technical regulations implied.
Examining Red Bull’s rear wing
The images from previous 2021 Grand Prix’s highlighted that the rear wing of the Red Bull was without a doubt experiencing some immoderate bending, moving backwards. This happened in order to reduce drag on straights and therefore increase straight-line speed.

(Source: F1technical.net)
How does it move rearwards?
Since a loaded aerodynamical element is impossible to be infinitely stiff, a small amount of deformation is unavoidable. Otherwise, the wing would be so brittle, eventually snapping and causing drivability issues, essentially creating a risk to safety for the drivers. This is where aeroelasticity materializes. In F1, aeroelasticity studies the way aerodynamical forces (pressure and friction) interact with an elastic body which is situated in airflow.
The way excessive aeroelasticity occurred for Red Bull is that the upper endplates and the upper rear wing were allowed by their mountings to slightly rotate backwards (under certain loads). These made the back of the rear wing tilt downwards which resulted in a reduced frontal area. To be technically specific, the formula of drag force is

(where: ρ=density of the fluid, v=speed of the object moving in the air, A= area of the object facing the air, and CD= drag coefficient). Based on its definition, a reduced frontal area translates to less drag, a massive advantage at high speeds, especially on the straights.
With this tilt happening, the wing’s angle of attack (= angle at which an aerofoil passes through air) was also reduced. A decreased angle of attack does not deviate the airstream as much and causes a loss in downward pressure on the top of the aerofoil (also known as positive pressure which causes the downforce effect), relating to the air velocity reduction over the shorter side of the aerofoil.
This phenomenon can be explained by looking into the physics behind downforce. Imagine an aerofoil which lies in airflow: curved streamlines form around it. Further away from the aerofoil, streamlines begin to straighten out. Where the streamlines are horizontally straight, the air pressure is equal to the atmospheric pressure. However, the closer the streamlines get to the top part of the aerofoil, the pressure increases gradually (= pressure gradient). On the bottom side of the aerofoil, the exact opposite occurs: the air pressure of the curved streamlines closer to the aerofoil is lower than the atmospheric pressure of the horizontally straight streamlines further below. Consequently, the pressure force acts downwards, hence the name ‘downforce’.
On the flipside, low run speeds are usually related to tight corners and/ or to winding sectors. Here, a bigger rear wing is more effective with producing downforce, while drag does not play as big of a role as on straights. Under these circumstances, the wing maintains its approximate initial shape and performs its job to produce immense amounts of downforce. This means the rear flexiwing alone fulfils 2 roles almost completely: shallow and aerodynamical at fast paces along with bulky and downforce efficient at low speeds. On some tracks the advantage could be around 0.1s/ lap and on others, it could be around 0.5s/ lap, depending on track specs.
What about a flexible front wing?
Onboard cameras behind the front wing displayed the flexibility of Mercedes’ front wing, similar to Red Bull’s rear wing.
A front wing’s relation with airflow
Looking closely at the relationship of the front wing with airflow, a Formula One car has 3 major points where downforce is hugely produced: the front wing, bargeboards and the rear wing. The front wing is the most important because it is the first element to come in contact with the airflow, consequently shaping it around the car and dictating the aerodynamics lying behind it.
Comparable to the way the rear wing tilts backwards, same takes place for the front wing. It deflects under aerodynamic load, creating less drag when less downforce is needed and coming back to its initial shape under breaking (where downforce is important for planting the car and therefore being able to approach the corner in the most efficient way possible).
A feature of Formula One cars are that besides skin friction drag and form drag, induced drag also acts on their surface. Induced drag is only characteristic for objects which produce downforce. Downforce is the product of a higher pressure above the aerofoil and a lower pressure below it (and the aerofoil is at a negative angle of attack). The air’s tendency is to ‘spill’ from the high-pressure side over to the low-pressure side on the bottom.

(Source: rapid-racer.com)
Why? Because pressure is the permanent oscillation of air particles which ricochets into other air particles, so higher pressure means more energy (and more force). The higher-pressure region holds more energy, so it travels over to the lower pressure area in order to balance out the energy levels. This is how wingtip vortices are created. Most often, these are aerodynamical challenges for Formula One cars. Solution: using the same concept of a reduced effective angle of attack, the result is the cutback of wing tip vorticity.
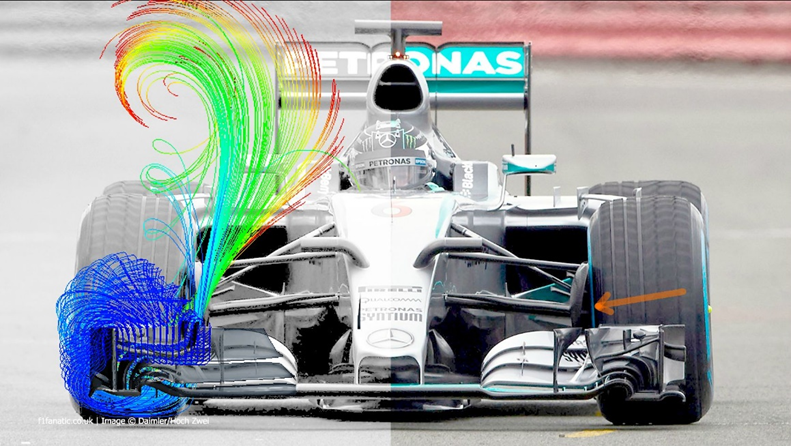
Diagram to demonstrate wingtip vorticles (Source: insideracingtechnology.com)
Which one of the two aeroelastic wings gave more of a performance boost is hard to analyse looking back especially as now, only the aeroelastic rear wing has been banned.
Comments